1. 서 론
2. 전통적 해양 입자유기탄소 순환 연구
3. 퇴적물 트랩을 이용한 해양 입자유기탄소 연구
3.1 퇴적물 트랩을 이용한 침강 입자 시료 채취
3.2 퇴적물 트랩 연구 현황
4. 방사성탄소동위원소와 재부유 퇴적물에 관한 고찰
4.1 MICADAS(Mini radioCarbon Dating Systems)를 이용한 방사성탄소동위원소 분석
4.2 재부유 퇴적물의 중요성
4.3 재부유 퇴적물의 기원
5. 맺음말
1. 서 론
심해(bathypelagic zone, 수심 >1000 m)는 해양에서 가장 거대한 생태계이자, 산업혁명 이후 대기 중 이산화탄소의 25% 가량을 흡수(연간 약 2.3 PgC), 제거하는 활동적인(active) 탄소 저장고이다(Sabine and Feely, 2007; IPCC, 2013). 또한 탄소를 대기와 해양으로부터 장기적으로(geological time scale) 격리시키고 저장하는 해저 퇴적물과 맞닿아 경계를 이루는 곳이다(Honjo et al., 2014). 이러한 중요성에도 불구하고, 지구상의 다른 탄소 저장고들에 비해 해양 심층 탄소 순환의 기작과 그 연결 고리에 대해서는 많이 알려져 있지 않다. 퇴적물 트랩(sediment trap)은 해저면으로 이동하는 침강 입자의 양(flux)과 종류(composition)에 관한 다양한 정보를 줄 수 있는 주요 도구들 중 하나이다. 해양 심층으로 침강하는 유기탄소는 이전에 알려졌던 것 보다 더 복잡한 형태이며, 시공간적으로 다양한 기원을 가지고 있다고 최근 연구에서 밝혀지고 있다(Druffel and Williams 1990; Eglinton et al., 2002; Goni et al., 2005; Hwang et al., 2010; Blattmann et al., 2018a; Kim et al., 2020a). 특히, 입자상유기탄소(POC: Particulate Organic Carbon)의 방사성탄소동위원소(14C) 분석을 통해 전체 침강 입자 중 재부유된(resuspended) 오래된 입자유기탄소가 차지하는 비율이 고전적인 생물학적 탄소 펌프(BCP: Biological Carbon Pump)에서 추정하는 것보다 훨씬 클 수 있음을 발견하였다(Hwang et al., 2010; Kim et al., 2020a). 이 논문에서는 이러한 발견에 핵심적인 정보를 제공해 준 퇴적물 트랩을 이용한 침강 입자 시료 채취와 방사성탄소동위원소 연구에 관하여 설명하였다. 또한 이를 이용하여 재부유 퇴적물의 중요성을 연구한 선행 논문들을 소개함으로써 국내 학자와 학생들의 관심을 불러 일으키고자 하였다.
2. 전통적 해양 입자유기탄소 순환 연구
해양은 대기 중 이산화탄소 농도를 조절하고, 대기와 해양 간 탄소의 균형을 맞추는 등 전지구적 탄소 순환에 있어 중요한 부분을 차지하며, 다양한 기작들을 통해 지구 기후에도 영향을 미친다(권과 조, 2013; Sigman and Haug 2003; Ridgewell and Arndt, 2015). 대기와 해양 간의 이산화탄소 교환에 영향을 미치는 주요한 인자 중 하나인 생물학적 탄소 펌프는 해수 표층의 유광대에서 용존무기탄소(DIC: Dissolved Inorganic Carbon)가 식물플랑크톤의 일차 생산을 통해 입자유기탄소의 형태로 변환되어 표층 탄소 저장고와의 탄소 교환이 어려운 수심 및 해저면으로 침강하는 일련의 과정을 일컫는다(Fig. 1, Volk and Hoffert, 1985).
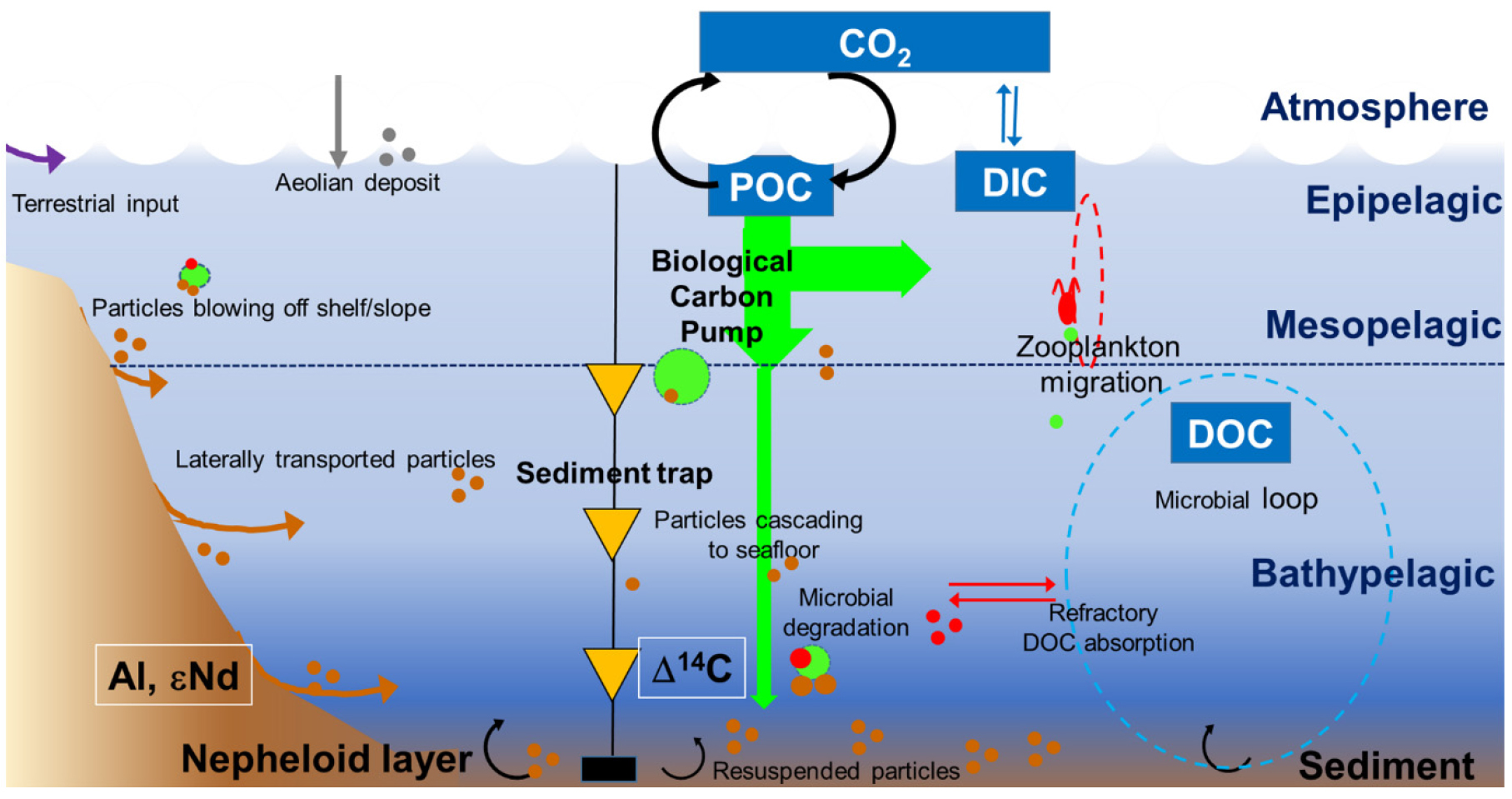
Fig. 1.
Schematic of the oceanic carbon distribution and sediment trap study. Sinking POC intercepted by sediment traps deployed in the deep ocean may reflect contributions from different sources/processes. Fresh POC shown as green colors and other potential POC sources are shown as gray (aeolian deposit), purple (terrestrial input), brown (lateral transport, resuspended), and red (DOC absorption), respectively.
표층해수층(epipelagic zone, 수심 <200 m)과 중층(mesopelagic zone, 또는 약광층, 황혼 지대(twilight zone), 수심 약 200-1000 m)에서는 동물플랑크톤이 입자유기탄소의 재광물화(remineralization)와 일주기 수직 이동(diel vertical migration)을 통해 생물학적 탄소 펌프 작용에 있어 핵심적인 역할을 한다(Buesseler et al., 2007a; Giering et al., 2014; Kelly et al., 2019; Cavan et al., 2019). 반면 비교적 고요한 환경인 심해에서는 용존 산소의 감소와 함께 미생물로 인한 재광물화(microbial degradation)와 중력에 의한 입자의 침강이 주를 이룬다(Fig. 1, Herndl and Reinthaler, 2013; Honjo et al., 2008, 2014).
중층에서 심층으로의 탄소 이동은 다양한 형태로 나타나며, 용승같은 물리적 기작(Zhang et al., 2019; Kim et al., 2020b), 수온이나 용존 산소 농도, 산성도와 같은 화학적 환경(Marsay et al., 2015; Honda et al., 2020), 생태계 구조(Ran et al., 2015), 그리고 입자유기탄소와 결합하여 빠른 침강을 돕는 역할을 하는 광물질(ballast mineral: Armstrong et al., 2002; Francois et al., 2002; Honda and Watanabe 2010; Le Moigne et al., 2014) 등 서로 다른 요인들에 복합적으로 영향을 받는다(Cram et al., 2018; Conte, 2019). 생물학적 탄소 펌프를 통해 심층으로 이동한 침강 입자는 먹이망을 따라 이동함으로써 해양의 생물 다양성을 유지하는 데 필요한 기본 에너지원을 제공하며, 이들은 해양 표층 환경의 환경 특성에 관한 정보를 해저면으로 이동시키는 역할을 하기 때문에, 침강 입자에서 기인한 퇴적물 기록을 통해 과거 표층 해수의 생산성이나 환경을 추측할 수 있다. 따라서 퇴적물 트랩을 이용하여 침강하는 입자(유기물과 생물의 사체 및 배설물 등)를 채취하고, 이를 연구함으로써 입자유기탄소의 침강 속도, 양과 종류, 기작, 재광물화, 용존유기물과 부유입자유기물의 생성 등 생지화학적 입자들의 시공간적 다양성을 파악하는 것은 해양 탄소 순환을 이해하는 데 있어 핵심적인 부분이다(Fig.1).
3. 퇴적물 트랩을 이용한 해양 입자유기탄소 연구
초기에 과학자들은 해양 심층에서는 굉장히 작은 크기의 입자들이 천천히 해저면으로 침강할 것으로 예상했다. 이후 퇴적물 트랩 연구는 수심에 따른 침강 입자 유기물의 양과 성분, 시공간적 변화를 이해하는 데 필요한 정보를 제공하였다(Lee et al., 1998; Honda et al., 2000; Fischer et al., 2003; Honjo et al., 2014; Wakeham et al., 2014). 최근 연구들에 따르면 훨씬 큰 크기의, 다양한 입자들이 침강하고, 이들의 침강 속도는 하루에 약 수백 미터 정도로 수십 일에서 수 개월에 걸쳐 해저에 도달하는 것으로 밝혀졌다(Honjo, 1982; Conte et al., 2001; Berelson, 2002; Riley et al., 2012).
최근에는 여러 관측 장비와 자동화된 센서들, 발달된 인공위성 측정과 더불어 모델 기반의 연구가 함께 진행되어 생물학적 탄소 펌프의 양과 이를 조절하는 요인들, 표층 탄소의 이동 효율 등에 관한 연구가 다양하게 진행 중이다(Lutz et al., 2002; Dunne et al., 2007; Gehlen et al., 2006; Siegel et al., 2014; Lima et al., 2014; Cram et al., 2018). 하지만 아직까지도 퇴적물 트랩은 심층 침강 입자에 관해 보다 직접적이고 핵심적인 시료와 정보를 제공하는 데 주축이 되는 도구이다.
3.1 퇴적물 트랩을 이용한 침강 입자 시료 채취
퇴적물 트랩은 계류 선에 추를 달아 해저면에 고정시키거나(bottom tethered, moored trap), 부이(buoy)에 부착하거나(surface tethered trap; Peterson et al., 2009), 부유시키는(freely drifting neutrally buoyant sediment trap; Buesseler, 1991) 등 계류 시간과 목적에 따라 단기/장기, 표층/심층 용의 다양한 종류가 있으나(Baker et al., 2020), 이 논문에서는 주로 심층에 장기적으로 계류하는 퇴적물 트랩 연구에 관하여 다루고자 한다.
최초의 해양 퇴적물 트랩은 1978년 버뮤다(Bermuda)의 3200 m 수심에 계류되었다. 이후 퇴적물 트랩은 여러 형태로 개발되었는데, 최근 널리 상용되고 있는 퇴적물 트랩은 McLane Research Laboratories (https://mclanelabs.com/sediment-traps/), Nichiyu Giken Kogyo Co. Ltd (https://www.nichigi.co.jp/en/en_products/en_nigkocean.html), SARL Technicap (https:// www.technicap.com/products/sediment-trap)사의 제품 등이 있다.
심층에 설치하는 장기 시계열 퇴적물 트랩은 수십년 간 해양의 입자상 유기물과 관련된 생물 기원 물질들의 수직적 이동을 이해하기 위한 주요 도구 중 하나로 사용되어 왔다. 해양 상층(>500 m)에 계류한 퇴적물 트랩은 수심 1-2 km에 계류했을 때에 비해 시료가 덜 채취되는 등 시료 채취 효율성에 있어 몇 가지 문제점이 있다고 알려져 있는 반면(Buesseler et al., 2007b; Gardner, 2000), 해류의 영향이 비교적 적은 심층 퇴적물 트랩은 최종적으로 해저로 전달되는 입자 수송에 걸리는 시간이나 침강 입자의 양과 성질 등에 대한 정보를 제공하는 중요한 도구로 활용되고 있다(Honjo et al., 2008).
해양 심층으로 침강하는 입자의 양은 표층에 비해 적기 때문에 우리가 흔히 접할 수 있는 퇴적물 트랩은 입자를 많이 모을 수 있도록 깔때기(conical, funnel), 혹은 실린더(cylindrical) 형태를 띤다(Fig. 2). 보통 깔때기 모양의 퇴적물 트랩은 실린더형 트랩에 비해 침강 입자를 모으는 효율이 떨어진다고 알려져 있는데(Bale, 1998; Ducklow et al., 2015; Baker et al., 2020), 입구가 넓기 때문에 해류로 인해 입구 주변에 작은 소용돌이(swirl)가 생기고, 침강해 내린 작은 입자들이 다시 쓸려 나가기 때문이다(Fig. 2, Gardner, 1980; Butman 1986; Gust et al., 1996). 이렇게 난류(turbulence)로 인해 입자가 부유하는 것을 최소화하고(Buesseler et al., 2007b) 큰 생물체나 입자가 트랩 내부로 들어와 입구가 막히는 것을 방지하기 위해 트랩의 입구 상부에는 벌집 모양의 뚜껑(baffle)을 덮는다(Fig. 2).
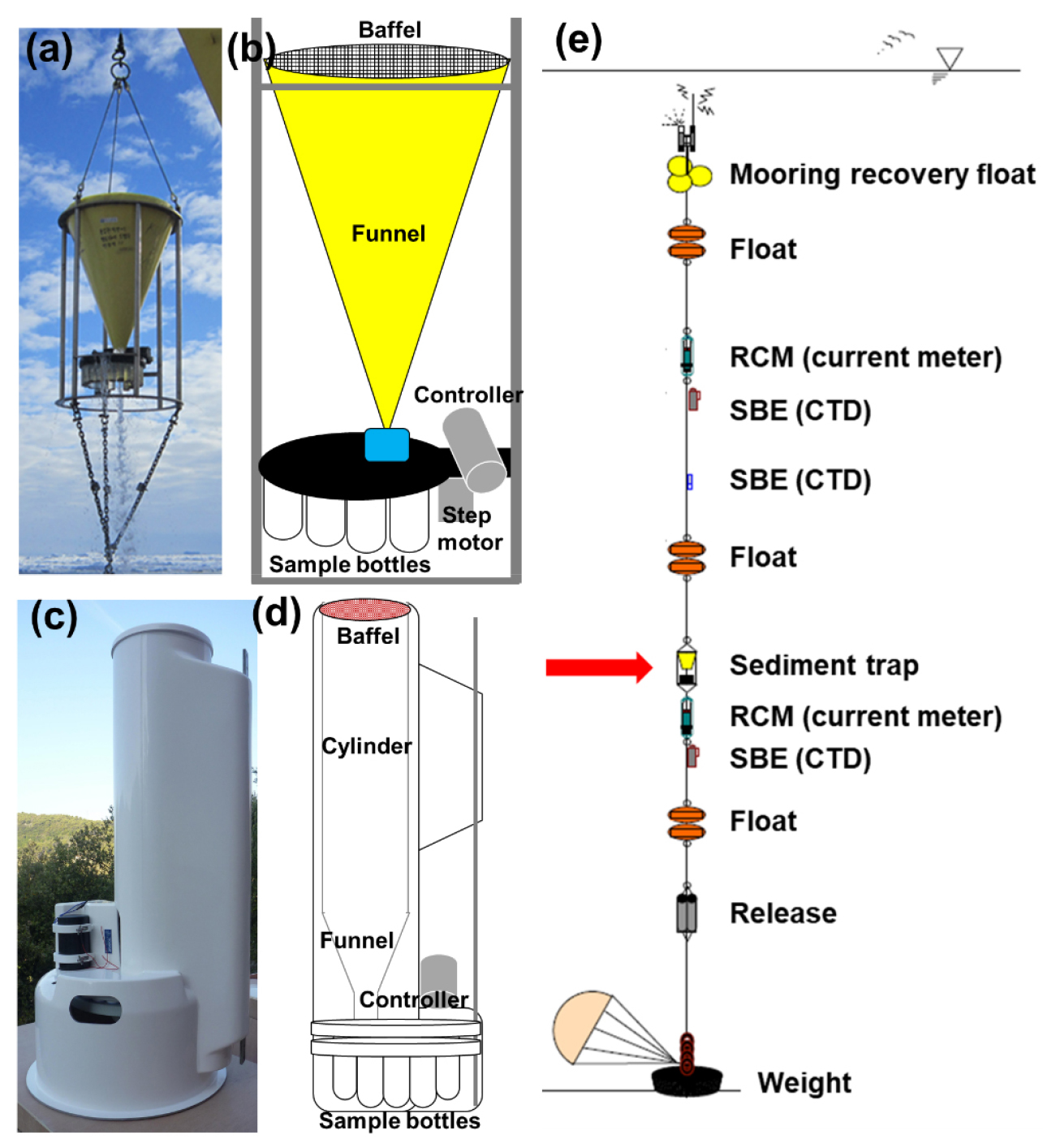
Fig. 2.
Conical sediment trap (McLane PARFLUX Mark 78G; figure courtesy of Minkyoung Kim) (a) with sketch and names of each parts (b), and cylindrical sediment trap (PPS-3/3, Technicap; figure from https://www.technicap.com/products/sediment-trap) (c) with sketch and names of each part (d, redrawn from Heussner et al., 1990). Layout diagrams of mooring line serviced by KOPRI (redrawn from Lee, 2012) is shown and the sediment trap is marked with an arrow (e).
깔때기 아래에는 미리 지정해 둔 시간에 맞추어 트레이(tray)를 회전시키는 제어장치(controller)와 트레이가 부착되어 있는데, 그 아래 약 21-26개 시료 채취병(bottle)을 부착할 수 있어서 프로그래밍 해 둔 시간에 따라 트레이가 자동으로 돌아가며 시료 채취병이 교체된다. 이러한 과정을 통해 시계열(time-series)의 침강 입자 시료를 모을 수 있다. 보통의 경우, 해양 표층의 일차 생산 등과 비교하여 침강 입자의 계절적 변동 등을 파악하기 위해 1년 가량을 주기로 계류 선과 퇴적물 트랩을 회수, 재계류하며, 해빙의 농도가 적은 시기에만 연구선의 접근이 제한적으로 가능한 극지 해역의 경우, 회수와 재계류로 인해 식물플랑크톤의 대번성 시기에 시료 채취를 하지 못하는 문제가 있어 2년을 주기로 계류하기도 한다. 더 자세한 사항은 Honjo et al.(1980)을 참고하기 바란다.
계류 전, 퇴적물 트랩의 내부는 보존제와 해수로 채우는 것이 일반적이다(Knap et al., 1996). 현장에서 계류 수심의 해수를 직접 채수하여 사용하는 것이 가장 이상적인데, 이것이 여의치 않다면 필터한 표층 해수(GF/F, φ=0.7 μm) 또는 실험실에서 제작한 인공 해수를 사용한다(35 PSU). 여기에 주변 해수보다 비중을 높게 만들어주기 위해 약간의 염(1L 당 5 g 가량의 NaCl)을 추가한다(Goto et al., 2016). 보존제에는 주로 포르말린(CH2O), 염화수은(HgCl2)이나 아자이드화나트륨(NaN3)이 사용된다. 보존제의 종류가 특정 분석에 영향을 미치기도 하므로, 퇴적물 트랩 계류에 앞서 분석 목적에 맞는 보존제를 선별하여 사용하는 것이 중요하다. 포르말린은 대부분의 분석 목적에 잘 맞고 가장 효과적인 보존액으로 알려져 있어 널리 사용된다(Knauer et al., 1984). 하지만 포르말린을 사용할 경우 생물 시료의 DNA 분석이 불가능하고(Kim et al., 2019b; Sano et al., 2020), 포르말린이 탄소를 포함하고 있기 때문에 탄소동위원소를 분석할 때 주의가 필요하다(Honda et al., 2000). 포르말린과 함께 염화수은도 입자유기탄소를 보존하는 데 매우 효율적이라고 알려져 있어 많이 사용된다(Wakeham et al., 1993; Goto et al., 2016). 염화수은과 아자이드화나트륨은 보존액 자체에 탄소를 포함하고 있지 않아, 탄소 분석에 많이 사용되었지만, 미량 금속 분석이나 점토 광물 분석을 할 때 주의해야 한다는 단점이 있다(Lee et al., 1992; Blattmann T.M., personal communications).
계류 선에는 퇴적물 트랩과 함께 부가적으로 기울어진 정도를 관측하기 위한 기울기 센서(Tilt sensor), 해류나 수층의 물리화학적 환경 변화를 파악하기 위한 ADCP(Acoustic Doppler Current Profiler)나 유속계(RCM; Rotor Current Meter), 수온/염분 센서계 등을 부착하여 추후 자료 해석에 활용한다(Fig. 2). 퇴적물 트랩을 비롯한 여러 계류 장비를 회수할 때에는 계류 선에 부착하였던 음향 이탈기(Acoustic release)를 이용한다. 연구선에서 음향 신호를 보내면 이탈기의 장치가 작동하여 해저의 추에서 상부 선이 분리되고, 계류 선과 장비들은 부이에 의하여 해수면으로 부상하게 된다.
퇴적물 트랩을 회수한 후에는 약 4°C 정도에 냉장 보관하며, 분석 전에 잠시 세워 두어 입자들이 잘 가라앉도록 한다. 퇴적물 트랩 시료는 다른 해양 시료에 비해 상대적으로 양이 적기 때문에 연구자들 간에 시료 배분 및 필요한 분석에 관한 사전 논의가 필요하며, 다양한 분석을 위해 여러 개로 소분하여 사용한다. 큰 크기의 동물 플랑크톤이나 크릴 등은 따로 보관하고, 나머지 시료는 1 mm 크기의 체(mesh)를 사용하여 큰 입자를 거른 후, 시료 분할기(Wet Samples Divider, McLane Research Laboratories, Inc., MA)를 이용하여 5~10개로 소분한다. 가장 우선적으로 시료의 기본 지화학분석(총입자량(Total Mass Flux), 유기탄소 및 무기탄소 함량, C/N 비 등)을 실시한 후, 나머지 시료를 기타 필요한 분석에 사용하고, 만일의 경우를 위해 시료의 일부는 보관해 놓는 것이 일반적이다. 보다 자세한 사항은 JGOFS 설명서(Joint Global Ocean Flux Study Protocol)에 잘 소개되어 있다.
3.2 퇴적물 트랩 연구 현황
전세계적으로 태평양, 대서양, 그리고 남극과 북극해 등지의 몇몇 정점에서는 장기 퇴적물 트랩 연구가 진행되어 왔다(Fig. 3; Table 1). 전지구적 퇴적물 트랩 연구 결과와 관련 정보는 JGOFS (http://usjgofs.whoi.edu/mzweb/ data/Honjo/sed_traps.html)나 PANGAEA (https://www.pangaea.de/) 웹사이트에서 얻을 수 있으며(Kim et al., 2020a), 정점에 따른 자세한 연구 결과는 각각의 참고문헌에 보다 상세히 소개되어 있다. 이 논문에서는 각 대양 별 대표적 장기 시계열 퇴적물 트랩 정점과 우리나라의 연구 정점들을 소개하였다.
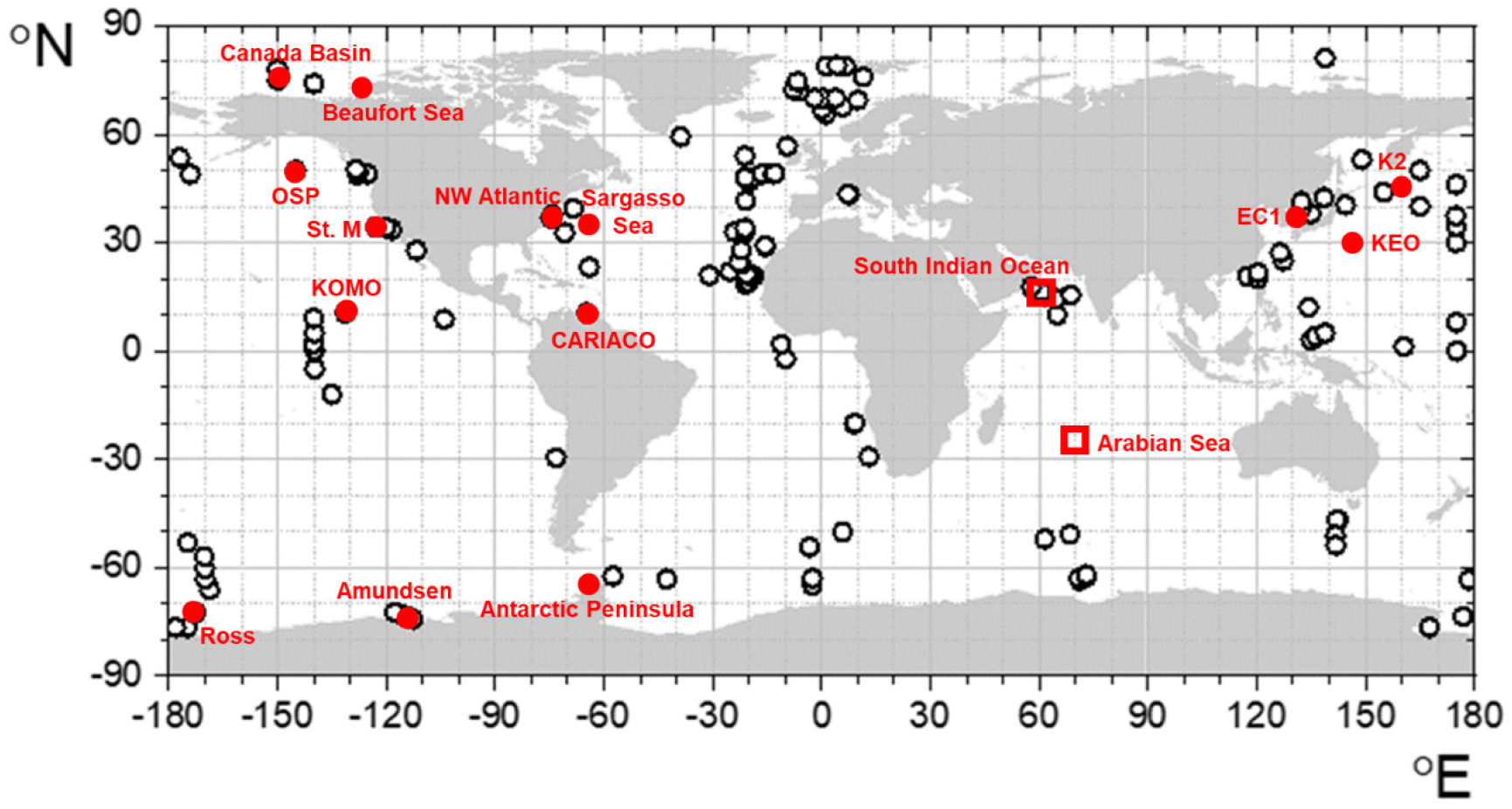
Fig. 3.
Locations where sediment traps were deployed (empty circle, redrawn from Kim et al., 2020a). Stations mentioned in this review paper shown in closed circle with station name. Two sites from the Indian Ocean are shown with open boxes, as multiple sampling was done in the locations.
Table 1.
Global long-term sediment trap mooring locations, water depth, trap depths, sampling periods, and representative references with related projects
Region, site | Lat (°) | Long (°) | Water depth (m) | Trap depths (m) | Periods (yr) | References | Project |
Pacific | |||||||
OSP | 50 | -145 | 4220 | 200, 1000, 3500-3700, 3800 | 1982-2006 | Timothy et al., 2013 |
Ocean Station Papa, NOAA https://www.pmel.noaa.gov/ocs/Papa |
St. M | 34.8 | -123 | 4100 | 3450 | 1993-1998 | Hwang et al., 2004; Smith et al., 2017 | Station M, NSF |
KEO | 32.3 | 144.6 | 5900 | 4900 | 2014-2018 | Honda et al., 2018; Anderson et al., 2020 | Kuroshio Extension Observatory, JAMSTEC, NOAA |
K2 | 47 | 160 | 5200 |
150, 540, (1000), 4810 | 2005-2011 | Honda et al., 2020 | K2S1 project, JAMSTEC |
KOMO | 10.5 | -131.2 | 5000 | 4950 | 2003-2013 | Kim et al., 2011; 2014; 2019 | Eastern Tropical Pacific, KIOST |
Ulleung Basin, EC1 | 37.63 | 131.36 | 2370 |
500(300), 1000, 2000 | 2011-present | Kim et al., 2017; 2020b | East Asian Seas Time-Series (EAST), KIOST http://east.snu.ac.kr/main.php |
Atlantic | |||||||
Sargasso Sea | 31.8 | -64.16 | 4500 | 500, 1500, 3200 | 1978-present | Conte and Weber, 2014 |
Bermuda Ocean Flux program https://www.mbl.edu/ecosystems/conte/ofp/ |
Cariaco Basin | 10.5 | -64.66 | 1400 | 152, 225, 410, 810, 1210 | 1995-2017 | Muller-Karger et al., 2019 | CARIACO Ocean Time-Series program http://www.imars.usf.edu/cariaco |
NW Atlantic | 39.5 | -68.35 | 3050 | 1000, 2000, 3000 | 2004-2008 | Hwang et al., 2009; 2017; 2020 | WHOI, https://scienceweb.whoi.edu/linew/index.php |
Indian | |||||||
Arabian Sea | 3.3~16.18 | 60.3~89.13 | 2267-3995 | 893-3189 | 1989-1998 | Ramaswamy and Gaye, 2006 | Bay of Bengal Process Study |
South Indian Ocean | -21.25~-27.80 | 68.59~73.89 | 3100-4000 | 659-3479 | 2014-2018 | Harms et al., 2021 | INDEX (Indian Ocean Exploration) program |
Arctic | |||||||
Canada Basin | 74~78 | -140~-150 | 3518~3825 | 2050, 3100, 3750 | 2004-2011 | Hwang et al., 2008; 2015 | WHOI, NSF |
Beaufort Sea | 71.5 | -127 | 400 | 100, 210 | 2004-2006 | Forest et al., 2010 | Que´bec-Oce´an, CASES and ArcticNet |
Antarctic | |||||||
Amundsen Sea | -72~-74 | -113~-118 | 530~1057 | 400, 490 | 2011-2017 | Kim et al., 2015; 2019a; 2019b | KOPRI |
Ross Sea | -72.5~-76.5 | -172.5~-175.5 | 543~769 |
230, (493) 519, 719 | 1990-1992 | Dunbar et al., 1989 | USJGOFS-AESOPS (Antarctic Environment and Southern Ocean Process Study) program |
Antarctic Peninsula | -64.5 | -66 | 350 | 170 | 1992-2013 | Ducklow et al., 2008 | Long-Term Ecological Research Project (LTER)https://oceaninformatics.ucsd.edu/datazoo/catalogs/pallter/datasets |
태평양의 Ocean Station Papa (OSP, https://www.pmel.noaa.gov/ocs/Papa; 50°N, 145°W; 수심 4220 m; 1982-2006; Timothy et al., 2013) 정점은 인공위성을 이용한 연구가 시작되기 이전에 과거 해양 기후를 모니터링 한 가장 오래된 시계열 자료가 존재하는 정점이다. 연구자들은 3800 m 수심에 단일 트랩을 계류한 것에서 시작해 여러 수심에서 침강 입자 시료를 얻었고, 다양한 연구를 통해 총 침강 입자 및 여러 생물 기원 입자들의 양, 침강 속도, 일차 생산과 계절에 따른 변화 등을 고찰하였다(Wong et al., 1999; Cummins and Lagerloef, 2004). 캘리포니아 해류가 지나는 곳에 위치한 M 정점(St. M; 34.8°N, 123°W; 수심 4100 m; 1993~1998; Hwang et al., 2004; Smith et al., 2017)에서도 28여년 간 다양한 연구가 지속되었다. 특히 인공위성 관측과 글라이더, 해양 심층 모니터링 카메라 등을 다채롭게 활용하여 입자유기탄소의 생산과 퇴적에 관한 전반을 이해하고, 궁극적으로는 표층에서 심해에 이르기까지 탄소 순환을 고해상도로 모델링 하는 연구들이 진행 중이다(Smith and Druffel, 1998; Sherman and Smith, 2009; Huffard et al., 2020). 일본 잠스텍(JAMSTEC) 해양연구소를 중심으로 KEO 정점(Kuroshio Extension Observatory, 32.3°N, 144.6°E; 수심 5900 m; 2014~2018; Honda et al., 2018; Anderson et al., 2020)과 K2 (47°N, 160°E; 수심 5200 m; 2005~2011; Honda, 2020) 정점에서도 연구 해역의 물리, 화학, 생물학적 특성과 침강 입자를 다층 중장기적으로 해석하는 연구가 진행 중이다. 우리나라에서도 한국 해양과학기술원(KIOST)를 중심으로 망간단괴 광구 해역 내 장기 모니터링 정점 ETP (Eastern Tropical Pacific, Station KOMO; 10.5°N, 131.2°W; 수심 5000 m; 2003~2013; Kim et al., 2008; 2011; 2014; 2019)에서 퇴적물 트랩 연구를 진행하였으며, 침강 입자의 중장기 시계열 자료를 통해 이 해역의 탄소 순환 양상을 엘리뇨, 라니냐 등의 기후 변동과 함께 해석하였다. 동해의 울릉분지에서도 1994/96년, 그리고 2000년 초반 퇴적물 트랩 연구가 산발적으로 진행된 이후(Suk et al., 2002; Hong et al., 2008a, 2008b), 2011년부터 현재까지 퇴적물 트랩을 계류 중이다(http://east.snu.ac.kr/main.php; 37.63°N, 131.36°E; 수심 2370 m; Kim et al., 2017; 2020b). 퇴적물 트랩 정점에 인접한 OceanSITES 정점(EC1)의 물리 해양학 자료(Chang et al., 2002; Noh and Nam, 2018, https://www.seanoe.org/data/00470/58134/)를 활용하여 울릉분지 침강 입자의 양과 생지화학적 성질을 파악하고, 침강 입자의 기원과 계절적, 연년 변동을 규명하였다.
대서양의 사가소 해역(Sargasso Sea)에서는 1978년에서 현재에 이르기까지 가장 오래된 시계열 퇴적물 트랩 연구가 진행되고 있다(Bermuda Ocean Flux 프로그램; 31.8°N, 64.16°W; 수심 4500 m, Conte and Weber, 2014). 특히 이 해역에서는 다양한 생물, 물리학적 관측과 연구를 통해 해양 상층과 심층의 물질 이동, 해양의 중규모 물리 변동(ENSO (El Niño-Southern Oscillation)나 NAO (North Atlantic Oscillation)) 및 화학적 변화(해양 산성화)로 인한 침강 입자의 변화 등 다양한 연구를 진행 중이다. CARIACO Ocean Time-Series 프로그램(http://www.imars.usf.edu/cariaco; 10.50°N, 64.66°W; 수심 1400 m; 1995~2017; Muller-Karger et al., 2019)에서는 총 5개 수심에 설치한 퇴적물 트랩을 이용해 표층 일차 생산, 침강에서 최종적 퇴적에 이르기까지 다양한 연구를 진행하고 있다. 특히 이 해역은 입자의 퇴적 속도가 빠른 편이라 고기후 연구를 하기에 좋으며, 탄소의 생물학적 탄소 펌프 효율이 높아(해저면까지 약 2%의 침강 입자유기탄소가 이동) 탄소 저장고로서도 중요한 역할을 한다(Walsh et al., 1999; Hughen et al., 2004; Montes et al., 2012; Turich et al., 2013). 한편 우즈홀 해양연구소(WHOI)를 중심으로 2004년부터 4년 간, 북서대서양 정점 W(39.5°N, 68.35°W, 수심~3050 m)에도 퇴적물 트랩을 계류하였으며(Hwang et al., 2009; 2017; 2020), 근처에 위치한 Line W의 물리해양학적 관측 자료(Toole et al., 2011; Andres et al., 2016; Le Bras, 2018; https://dods.ndbc.noaa.gov/thredds/catalog/data/oceansites/ DATA/LINE-W/catalog.html)를 활용해 침강 입자의 수평적 이동(lateral transport)을 보다 체계적으로 파악하였다.
해빙이 녹는 여름철의 몇 개월을 제외하고는 접근이 어려운 극지역에서도 퇴적물 트랩을 이용한 연구는 그 활용도가 높다. 특히 지구 온난화에 따른 해빙의 감소, 개구부(Polynya)의 형성 등 변화가 빠르게 발생하고 있으며, 해빙에 덮인 해역에서는 인공위성을 이용한 일차 생산 측정이 불가능하기 때문에 극지 해양에서 탄소 순환을 이해하는 데 있어 퇴적물 트랩 연구는 핵심적인 정보를 제공한다. 현재까지 남극 주변부의 약 50개 정점에서 장, 단기 퇴적물 트랩이 계류되어 왔으며, 서남극 아문젠해(Amundsen Sea, Kim et al., 2015; 2019a; 2019b), 로스해(Ross Sea, Dunbar et al., 1989), 그리고 남극 반도의 LTER (Long-Term Ecological Research, Ducklow et al., 2008)정점 등에서 퇴적물 트랩을 활용한 연구가 진행 중이다. 북극해 캐나다 분지(Fig. 4; Canada Basin, Hwang et al., 2008; 2015)나 보퍼트해(Beaufort Sea, Forest et al., 2010)에서도 시계열 퇴적물 트랩을 이용하여 환경 변화에 따른 입자유기탄소의 순환 양상과 공급원에 대해 고찰하였다. 우리나라에서는 쇄빙선 아라온호를 활용하여 남극과 북극을 오가며 활발한 연구를 진행 중이다. 서남극 아문젠해 해빙역에서는 약 3년에 걸친 침강 입자 자료를 얻고, 이를 해빙의 농도와 비교하여, 해빙이 열리는 시기와 정도에 따라 입자유기탄소의 양이 연간 큰 차이가 있음을 발견하였다(Kim et al., 2019a). 북극의 척치해(Chukchi Sea)와 동시베리아해에도 2017년부터 퇴적물 트랩을 계류하여 침강 입자의 연변화와 이에 영향을 주는 요인을 파악하는 연구를 시작하였다(Kim et al., 2021). 이렇게 기후 변화에 민감하게 반응하는 극지 해양에서 퇴적물 트랩을 활용한 연구를 통해 탄소 순환의 변화를 정확히 감지하는 것은 미래 기후변화 예측에 중요한 자료를 제공한다.
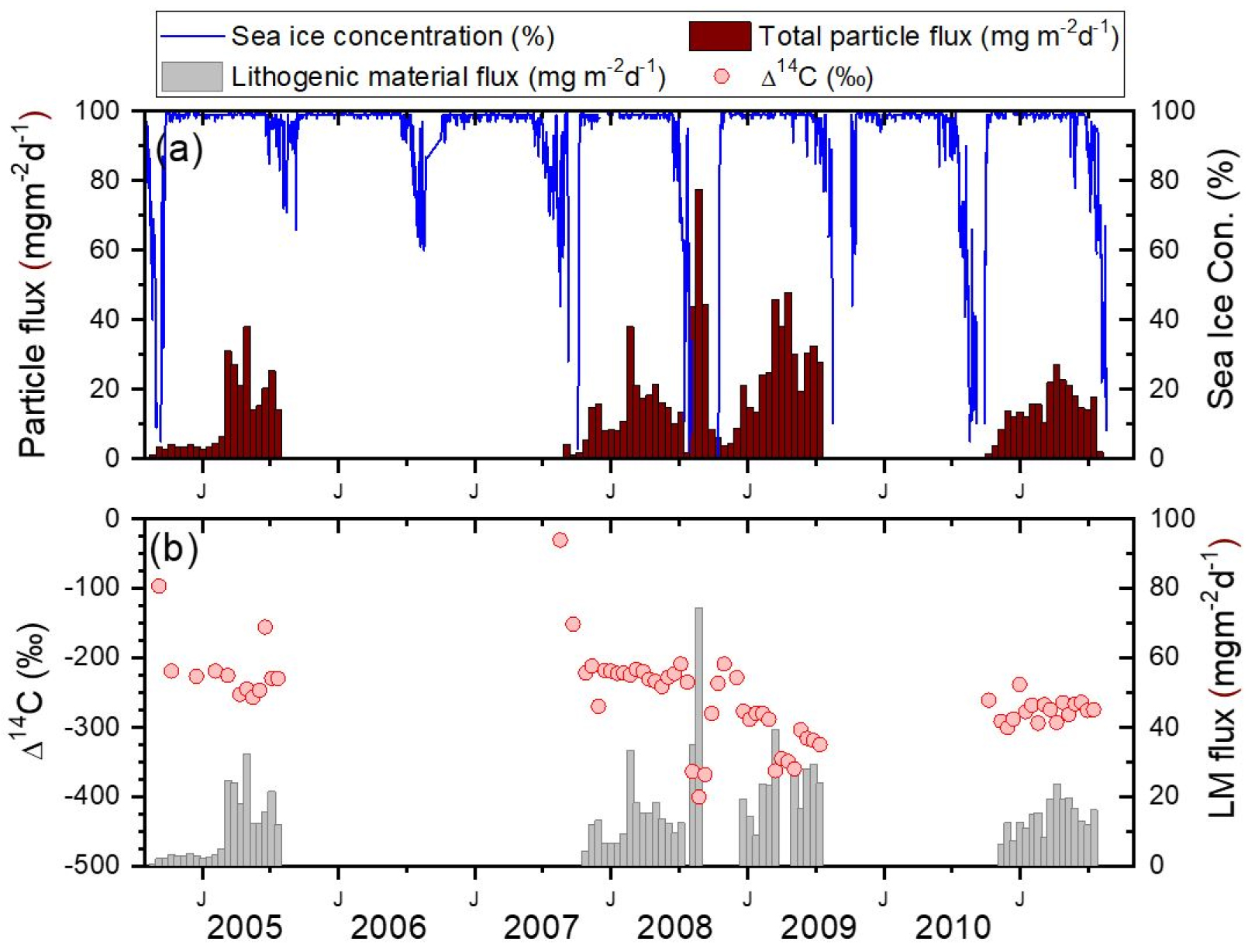
Fig. 4.
(a) Particle flux (bar) at 3100 m depth and sea ice concentration (line) in the Arctic Canada Basin. (b) 14C (circles) values and lithogenic material (LM) flux (bar) are also shown. Period where marked with orange box shows larger particle flux, larger LM flux, lower (aged) 14C values than in other years (modified from Hwang et al., 2015).
위와 같이, 우리나라의 퇴적물 트랩 연구는 미국을 중심으로 1970년대 말 시작된 연구들에 비해 시작은 늦었지만, 풍부한 인프라를 바탕으로 다양한 해역에서 퇴적물 트랩을 계류하며 국제적으로 높은 수준으로 발돋움하고 있다. 태평양과 대서양, 극지 해양에서 다양한 계류가 진행되어 온 데 비해, 인도양에서는 비교적 발표된 연구 결과가 적은 편이다(Fig. 3; e.g., Ramaswamy and Gaye, 2006; Harms et al., 2021). 향후 이사부호 등 해양 관측선과 개선된 퇴적물 트랩 장비를 이용한 국내 과학자들의 활약을 기대해 본다.
한편 보다 성공적인 장기 시계열 자료 획득을 위해서는, 시료 채취의 기술적 측면에서 겪고 있는 어려움을 해결하려는 노력도 수반되어야 한다. 동해 울릉분지에서는 약 10여년 간 퇴적물 트랩 연구가 지속되었음에도, Kim et al.(2020b)에 소개된 침강 입자의 시계열 자료는 여러 군데에 공백이 존재한다. 대부분 퇴적물 트랩 깔대기의 배출구가 막혀 시료 채취에 실패했기 때문인데(2012년 7-12월, 2013년 9-12월, 2014년 1-4월 등), 이를 해결하기 위해 기존의 깔때기형 트랩을 실리더형 트랩으로 교체하였다. 남극 아문젠해에서도 퇴적물 트랩의 트레이가 작동하지 않는 등의 이유로 시료 채취 성공률이 높지 않아, 퇴적물 트랩의 제작사를 교체하는 등 해결책을 모색하고 있다(Dr. Yang, Personal communication). 이렇게 퇴적물 트랩의 배출구가 막히거나(Miquel et al., 2011), 회전 트레이의 고장과 같은 기기적 결함(Harms et al., 2021)은 퇴적물 트랩을 이용한 여러 연구에서 발생하는 고질적인 문제이다. 따라서 퇴적물 트랩을 이용한 입자 채취 방법에 관한 보다 근본적이고 기술적인 연구(실험실과 현장에서의 유체역학적 실험과 모델을 통한 트랩 디자인의 보완 및 개발 등)가 수반되어야 할 것이다(Jürg 1996; Estapa et al., 2020).
4. 방사성탄소동위원소와 재부유 퇴적물에 관한 고찰
4.1 MICADAS(Mini radioCarbon Dating Systems)를 이용한 방사성탄소동위원소 분석
방사성탄소동위원소는 성층권에서 우주선에 의해 생성되어 약 5730년의 반감기를 거쳐 자연적으로 방사성 붕괴를 하여 그 양이 줄어든다(Godwin, 1962). 한편 20세기 중반, 핵실험의 결과로 방사성탄소동위원소는 “bomb-spike”라는 특이한 모양을 보이며 그 농도가 급격히 증가하였다가 원래의 값으로 회복되고 있다(Levin and Hesshaimer, 2000; Schuur et al., 2016). 다양한 탄소 저장고(대기권, 생물권, 지질권, 그리고 표층/심층 해수권)에서 발견되는 이러한 방사성탄소동위원소값의 차이는 서로 다른 체류 시간과 탄소 교환 속도를 반영하는데, 따라서 방사성탄소동위원소는 수십년에서 수만 년에 걸친 탄소 순환의 추적자 뿐 아니라 시공간적 변화를 이해하는 데 중요한 도구로 사용되어 왔다(McNichol and Aluwihare et al., 2007). 예를 들어, 대기 중의 bomb-spike는 보다 안정적인 탄소 저장고인 지질권이나 심해 쪽으로 천천히 이동하고 있기 때문에, 해양 수괴 순환이나 지역적 대기-해양 간의 탄소 교환을 이해하는 데 쓰일 수 있다(Graven, 2015; Toggweiler et al., 2019).
이 논문에서는 스위스연방공대(ETH Zurich)의 Laboratory of Ion Beam Physics (LIP)에서 제작하여 최근 널리 통용되고 있는 MICADAS (Mini radioCarbon Dating Systems; https://www.ionplus.ch/micadas) AMS (Accelerator Mass Spectrometry)를 이용한 방사성탄소동위원소 측정법을 소개하고자 한다. 전통적인 방사성탄소동위원소 측정 방법은 Hwang(2012)에 잘 소개되어 있다. MICADAS는 기존 AMS에 비해 기기 부피가 작고(3.2 m × 2.6 m × 2 m), 다양한 분석을 보다 간단히 하도록 개발하여 방사성탄소동위원소 분석의 실용성을 크게 향상시켰다(Synal et al., 2007; Wacker et al., 2010).
MICADAS를 이용하여 방사성탄소동위원소를 분석하려면 대략 탄소100μg이 필요한데, 퇴적물 트랩으로 채취한 침강 입자의 유기탄소 농도가 약 5% 라면 2 mg 정도의 건조 시료만으로도 분석이 가능하다(Haghipour, N., personal communication). 특히 스위스연방공대의 MICADAS는 원소 분석기(Elemental Analyzer, vario MICRO cube, Elementar), 안정동위원소 분석기(Stable Isotope Ratio Mass Spectrometer: IRMS, visION, Isoprime)와 결합된 형태로 이산화탄소 기체를 바로 이온화시켜 중간 시료 처리 과정(금속 촉매를 이용한 흑연화 등)을 단축시키고, 고체 시료로부터 곧바로 입자유기탄소의 농도(%)와 안정동위원소(13C), 방사성탄소동위원소 값을 한 번에 측정할 수 있어 분석에 필요한 시료량과 분석 시간을 크게 단축시켰다(Fig. 5; Synal et al., 2007; Wacker et al., 2010, 2013; McIntyre et al., 2017). 또한 EA-IRMS 기기를 이용해 분석을 하는 동안, 따로 탄산칼슘이나 앰퓰 크래커(ampoule cracker)를 이용한 AMS 분석이 동시에 가능하다(Fig. 5; Ruff et al., 2010; McIntyre et al., 2017). 입자상 시료 뿐 아니라 용존상 시료(용존무기탄소)의 전처리 과정 또한 간편화 되어 보다 효율적인 분석이 가능하다(Blattmann et al., 2018b; Casacuberta et al., 2020). 하지만 MICADAS를 이용한 분석은 오차가 대략 5‰ 이내로 기존의 흑연으로 환원시켜 분석하는 방법에 비해 떨어진다는(±2~3‰) 단점이 있다. 그렇지만 이는 침강 입자 시료의 기원 추적이나 bomb-signal 등을 보기에는 충분히 신뢰할 만한 값이며 정밀분석을 통해서는 1.5‰ 이내까지 오차를 줄일 수 있다. 즉, MICADAS의 분석 오차가 기존의 AMS비해 크긴 하지만, 다양한 시료의 전처리와 분석 과정이 빠르고 간편해 졌기 때문에 그 동안 비용적, 분석방법적인 문제로 분석 결과가 많지 않았던 방사성탄소동위원소 데이터 획득에 큰 긍정적 변화를 주고 있다.

Fig. 5.
Schematic of the EA-IRMS-AMS system at ETH Zurich. Samples are combusted in an EA, transferred to IRMS, and the gas is split by IRMS so 10% of the gas used for 13C and 90% for 14C analyses (modified from Ruff et al., 2010; Wacker et al., 2013; McIntyre et al., 2017).
특히 Timothy Eglinton교수의Biogeosciences group을 선두로 하여 스위스연방공대에서는 MICADAS를 이용한 블랭크바탕값(blank) 보정 및 분석법을 개발하고(Welte et al., 2018; Haghipour et al., 2019), 특정 유기화합물의 방사성탄소동위원소 분석을 간편화 하며(Feng et al., 2015; Hanke et al., 2017; Ishikawa et al., 2018; Gies et al., 2021), 다양한 해역에서 국제적 공동연구를 통해 다량의 방사성탄소동위원소 분석을 진행하고 있다(Bao et al., 2016; Lin et al., 2019; Yu et al., 2019). 이를 바탕으로 MOSAIC (Modern Ocean Sediment Archive and Inventory of Carbon; https://mosaic-eth.shinyapps.io/MOSAIC_Rshiny/)이라는 데이터 베이스도 구축 중이다(van der Voort et al., 2020). 향후 MOSAIC이 GEOTRACES나 GEODAP(Global Ocean Data Analysis Project)과 같은 전지구적 데이터 베이스로 자리잡게 되는데 있어 국내 연구자들도 다양한 방법으로 이바지할 수 있기를 기대한다. 이를 위해 장기적으로 가장 이상적인 방법은 국내 MICADAS 시스템의 구축이다. 현재 스위스연방공대 측에서 방사성탄소동위원소 분석을 위해서는 시료 1개 당 700스위스프랑(90만원) 정도의 비용이 필요하다. 그 외 AMS측정 장비를 보유한 미국 우즈홀 해양연구소(WHOI-NOSAMS; National Ocean Sciences Accelerator Mass Spectrometry)에서는 약 700미국달러(80만원) 정도, 국내 한국 지질자원연구원(KIGAM;https://gac.kigam.re.kr/sub0604)에서도 시료 당 분석 비용이 30~50만원 가량이 든다. 따라서 국내 해양 연구소에 MICADAS를 구축한다면 분석 비용의 절감, 분석의 효율성과 편리성, 그리고 분석 속도 향상에 크게 기여하여 방사성탄소동위원소를 활용한 우리나라 연근해 및 대양 연구에 중요한 자료를 제공할 수 있을 것이다.
4.2 재부유 퇴적물의 중요성
대양에서 생물학적 탄소 펌프를 통한 수직적 이동이 해저면 퇴적물의 주요 기원인 것과 달리, 연안과 대륙 사면부의 경우 강한 해류로 재부유된 입자들이 혼탁층(nepheloid layer)을 형성하며, 이들의 수평적 이동이 수층 입자의 상당 부분을 차지하기도 한다. 따라서 생물학적 탄소 펌프 외에도 혼탁층에서의 재부유와 입자들의 수평적 이동 등 역동적인 해양 저층의 기작을 이해하는 것은 해양 탄소 순환을 이해하는 데 있어 중요한 부분이다. 상당한 양의 오래된 유기물이 비생물기원 입자(Non-biogenic 또는 Lithogenic material (LM))와 결합되어 재부유나 수평적 이동 과정을 통해 해양 저층에 존재하고 있으나, 이에 대한 기작이나 생지화학적 중요성에 관한 부분은 아직 많이 연구되지 않았다. 알루미늄(Al)은 이렇게 해저에서 재부유되어 이동하는 입자들의 이동 경로나 양을 추적하기 위해 가장 널리 쓰이는 추적자 중 하나이다. 알루미늄은 지각을 구성하는 주요 원소로, 지각 내 8.23% 정도의 비교적 일정한 비율로 존재한다(Taylor and McLennan, 1985). 수층의 알루미늄은 대기를 통해 공급되기도 하지만, 해저면이나 대륙에 가까울수록 그 주요 공급원은 재부유된 퇴적물이다(Hwang et al., 2010; Kim et al., 2020a; 2020b). 전체 침강 입자 시료 중 재부유 퇴적물의 비율은 두 가지 방법으로 구할 수 있다. 첫번째로는 알루미늄의 농도에 12.15를 곱한 값(=100/지각 내 알루미늄의 존재비(8.23))을 사용하는 것이고(Honjo et al., 2000), 두번째로는 전체 침강 입자 중 생물학적 기원의 입자를 제외한 값(=100-오팔(Opal)-탄산칼슘(CaCO3)-입자유기물(입자유기탄소x1.88)을 사용하는 것이다. 여기에서 생물학적 기원의 입자들은 오팔, 탄산칼슘과 같은 해양생물의 껍데기(shell)와 입자유기물(POM: Particulate Organic Matter)을 포함한다. 입자유기물은 보통 입자유기탄소의 값에 1.88(Lam et al., 2011) 또는 2.5 (Thunell, 1998)를 곱한 값을 사용한다. 전자는 NMR (Nuclear Magnetic Resonance)로 측정한 식물플랑크톤의 생체 조성비(Hedges et al., 2001)이고, 후자는 CH2O 형태의 입자 유기물 중 탄소가 차지하는 원자량을 이용해 간단히 정한 값이다. 이렇게 구한 값을 비생물기원 입자라고 하며, 보통의 경우 위의 두 방법으로 유추한 비생물기원 입자의 양은 크게 다르지 않다(Kim et al., 2020a).
전지구적으로 약 150여개 퇴적물 트랩 정점의 연구 결과를 모아 그 중 비생물기원 입자의 양과 비율을 정리해본 결과, 재부유 퇴적물은 전체 침강 입자의 평균 25%를 차지함으로써, 퇴적물의 재부유가 우리 생각보다 해양 내부에서 널리 일어나는 중요한 현상임을 알 수 있었다(Kim et al., 2020a). 입자의 양이 많은 혼탁층 뿐 아니라 심해에서도 강한 에디(eddy)가 있는 경우, 퇴적물 재부유와 수평 이동하는 탄소의 영향은 전체 침강입자유기탄소의 약 4-11%로 무시할 수 없는 수준이며(Gardner et al., 2018; Kim et al., 2020a), 북극해 캐나다 분지를 비롯한 몇몇 해역에서는 비생물기원 입자가 전체 입자의 80%에 달하는 등 이러한 기작으로 인한 입자의 유입이 수직적 이동에 의한 것보다 많았다(Fig. 4, Hwang et al., 2008, 2015). 이는 전지구적으로, 수직적 탄소의 침강 뿐 아니라 수평적 이동과 재부유 퇴적물이 탄소 순환의 중요한 기작 임을 의미한다(Anderson et al., 1994; Jahnke, 1996; Bauer and Druffel 1998; Dunne et al., 2007; Hwang et al., 2010; Thomsen et al., 2017; Kim et al., 2020a).
대부분의 경우에, 침강입자유기탄소의 Δ14C 값은 표층 부유 입자의 Δ14C나 동시간대 표층 해양의 용존무기탄소의 Δ14C보다 낮게 관측되었다. 식물플랑크톤의 광합성으로 용존무기탄소에서 입자유기탄소로 전환되는 과정에서, Δ14C 값으로 표기되는 방사성탄소동위원소 값은 동위원소의 분별 작용(fractionation)의 영향을 제거한 값이며, 해수 표층에서 일차 생산을 통해 생성된 입자유기탄소의 침강 속도(10–100 m d−1)를 고려한다면(Honjo, 1982; Conte et al., 2001; Berelson, 2002; McDonnell and Buesseler, 2010; Riley et al., 2012), 침강 입자유기탄소의 Δ14C는 표층수의 용존무기탄소의 Δ14C 값을 반영해야 한다. 두 값 사이에 차이가 있다면 이는 다른 기원(육상이나 대기에서의 유입, 재부유되는 해저 퇴적물로부터 기원, 용존유기탄소의 흡착 등)의 유기탄소가 유입되었음을 뜻한다(Druffel and Williams 1990; Eglinton et al., 2002; Goni et al., 2005; Blattmann et al., 2018a).
그 중 이 논문에서 다루고자 하는 것은 재부유된 퇴적물에서 기원하는 입자유기탄소이다. 이들은 새롭게 침강하는 입자유기탄소에 비해 오래된 탄소들로, 비교적 낮은 Δ14C 값을 보인다(Hwang et al., 2008, 2010, 2017; Kim et al., 2020a, 2020b). Hwang et al.(2010)은 입자유기탄소의 Δ14C 값이 침강 입자의 Al%와 음의 상관관계를 보임을 발견하였는데, 이 관계는 오래된 입자유기탄소의 주요 기원이 재부유 퇴적물과 관련이 있음을 의미한다(Sherrell et al., 1998; Honda et al., 2000; Hwang et al., 2010; Kim et al., 2020a). 이후 Kim et al.(2020b)은 2010년 이후 분석된 연구 결과들을 더하여 해석을 시공간적으로 확대하고, 침강 입자 중 비생물기원 입자(점토성광물, 주로 aluminosilicate minerals)와 혼합된 입자유기탄소의 관계에 초점을 맞추어 추가 연구를 진행하였다. 특히 Hwang et al.(2010)이 사용한 알루미늄의 농도(=Al/(비생물기원입자+입자유기물+오팔+탄산칼슘)) 대신 입자유기탄소와 비생물기원 입자의 관계만을 이용하여(=비생물기원 입자/ 입자유기탄소) 침강 입자를 신선한 생물 기원 물질과 비생물기원 입자와 결합된 재부유 기원 물질로 구별하였는데, 이 값은 Al%를 사용한 기존의 그래프가 침강 입자와 퇴적물 간의 입자유기탄소 농도 차이로 인해 위로 볼록한 형태를 보였던 것과 달리(Fig. 6a, Hwang et al., 2010), 각 정점에서 입자유기탄소의 Δ14C와 직선형의 상관 관계를 보여 y=ax+b의 형태로 다양한 해석에 손쉽게 활용할 수 있게 되었다(Fig. 6b, Kim et al., 2020a). 이 직선의 y축 절편(b)은 비생물기원 입자의 농도가 0, 즉 대기나 강에서의 비생물기원 입자 유입이 없다고 가정할 때의 표층 용존무기탄소의 Δ14C의 값을 의미한다. 또한 각 직선의 기울기(a)와 방사성탄소동위원소 값의 끝성분(end-member; 주로 표층 용존무기탄소의 Δ14C와 표층 퇴적물의 Δ14C) 값을 이용하면, 재부유퇴적물 내 비생물기원 입자와 결합한 입자유기탄소의 비율을 얻을 수 있다. 이 비를 이용해 퇴적물 트랩으로 채취한 입자유기탄소 중 몇 %가 재부유 기원인지를 규명하였다. 자세한 설명은 Kim et al.(2020a)을 참고하기 바란다. 이 연구에서 다룬 연구 정점은 총 여섯 곳으로(Fig. 6에는 남중국해 정점이 추가됨), 이는 시료의 양이나 분석 비용의 문제 등으로 침강 입자유기탄소의 Δ14C 분석을 진행한 사례가 전지구적으로 많지 않기 때문이다. 최근 스위스연방공대에서는 MICADAS를 활용하여 북서대서양 정점에서 약 300여개의 입자유기탄소의 Δ14C를 추가로 분석하였으며, 국제 공동 연구를 통해 퇴적물 트랩 시료의 입자유기탄소의 Δ14C 자료의 시공간적 확장을 계획 중이다.
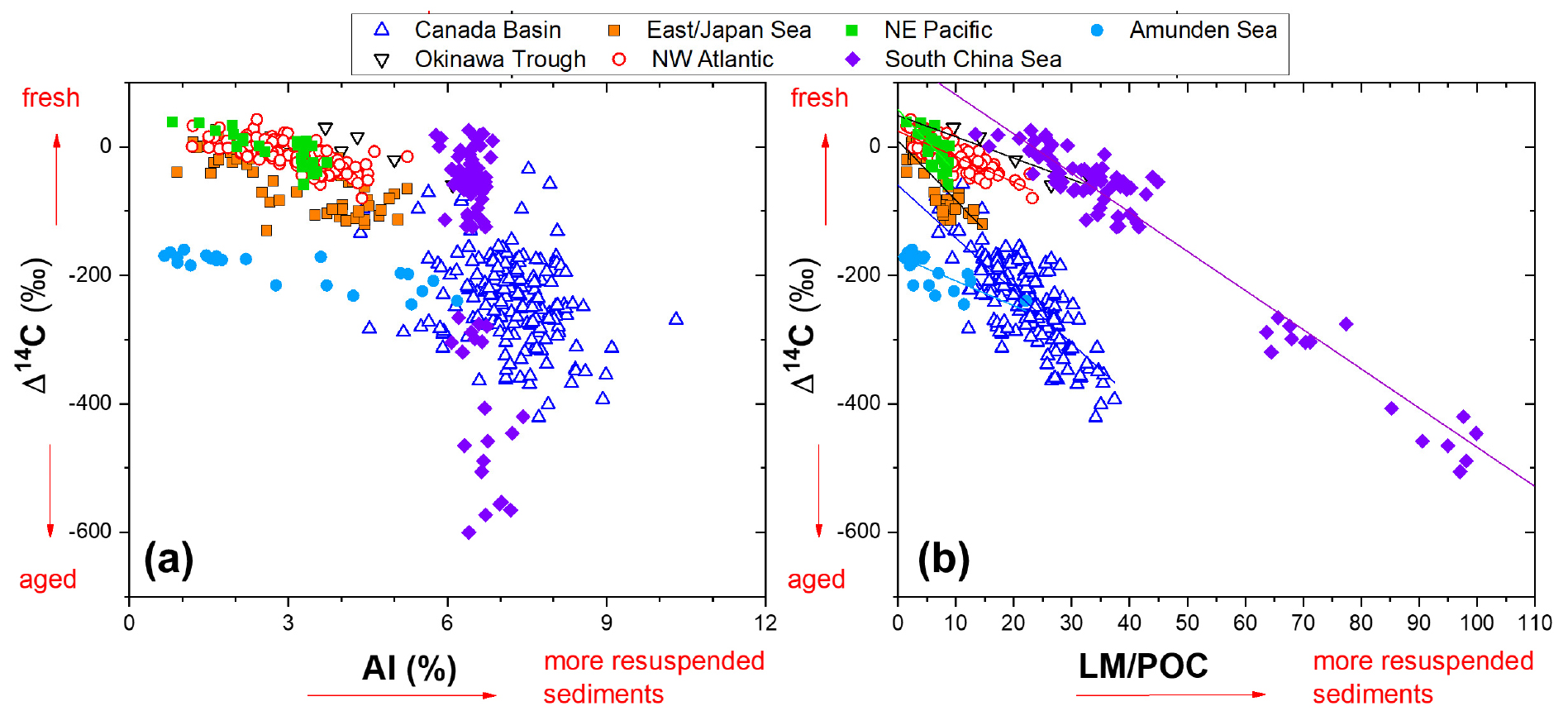
Fig. 6.
(a) Plot of 14C values of POC versus aluminum contents in sinking particles samples (modified from Hwang et al., 2010) and (b) plot of 14C values of POC versus the ratio of lithogenic material to POC (LM/POC) for sediment trap samples where both types of data exist (modified from Kim et al., 2020a and data from the South China Sea has been added from Blattmann et al., 2018a).
4.3 재부유 퇴적물의 기원
한편 여기에서 나아가, 재부유 퇴적물의 기원을 보다 자세히 파악하고, 재부유 기작을 규명하고자 하는 최근의 몇몇 연구를 간단히 소개하겠다.
첫째로, Al 외에도 표층 퇴적물의 기원 암석에 따라 네오디뮴 동위원소 값(εNd=[(143Nd/144Nd)sample/(143Nd/144Nd)CHUR -1] x10000; 여기에서 (143Nd/144Nd)CHUR =0.512638; Jacobsen and Wasserburg, 1980)이 다르다는 점을 이용하여 침강 입자 시료의 기원을 보다 정확히 추적하는 연구가 있다(Fig. 1). εNd 값은 특히 입자가 이동하는 중 해류에 의한 광물학적 분별 작용이 발생하지 않고, 입자 크기 등의 영향을 받지 않는다는 장점이 있다(Jonell et al., 2018). 지각의 εNd 값은 암석의 형성 시기와 종류에 따라 매우 다르며(Goldstein and Hemming, 2003), 이러한 동위원소비의 차이는 풍화나 침식에 의해 암석에서 떨어져 나온 비생물기원 입자에도 그대로 반영된다. 따라서 주변 퇴적물의 εNd 값의 공간적 차이를 통해 입자의 기원지 및 이동 궤적을 유추할 수 있다(Grousset et al., 1990; Hegner et al., 2007; Jeandel et al., 2007; Hwang et al., 2020). 한 예로, 고에너지 환경인 북서대서양 대륙 주변부의 침강 입자는 재부유 퇴적물과 오래된 유기물의 영향을 많이 받으며 그 주요 기원은 대륙붕과 대륙 사면을 따라 존재하는 혼탁층으로 추정된다(Hwang et al., 2009, 2017). Hwang et al.(2020)은 북서대서양 해저면 퇴적물의 εNd 값이 해역에 따라 5정도 차이가 나는 것을 이용하여, 침강 입자의 εNd 값을 분석해 입자의 이동 기작과 기원지를 보다 정확하게 유추하였다.
두번째로는 퇴적물의 재부유나 수평 이동 시 입자유기탄소의 분별 작용에 관한 연구이다. 이 또한 적은 양의 시료로 간단하고 빠르게 방사성탄소동위원소 분석이 가능한 MICADAS의 이점을 살린 연구로, 침강 입자 시료의 밀도 분별(density fractionation)을 통해 어떤 밀도와 나이의 퇴적물이 주로 재부유 되는지, 그리고 밀도 별 입자들의 방사성탄소동위원소 등 생지화학적 특성은 전체(bulk) 시료와 비교해서 어떠한지 등을 파악할 수 있다. 밀도 분별은 주로 토양(soil) 연구에서 사용하는 개념으로, 해양 침강 입자나 퇴적물에서는 활용 사례가 많지 않다(Bock and Mayer, 2000; Dickens et al., 2006; Wakeham et al., 2009). Wakeham et al.(2009)이 해양 퇴적물에서 1.6 g cm-3 이하, 2.5 g cm-3 이상, 그리고 그 사이의 밀도로 퇴적물을 분별하여 각각의 유기탄소 함량과 탄소동위원소 등을 측정한 연구에 따르면, 밀도가 큰 퇴적물일수록 유기탄소 함량이 작고, 오래된 유기탄소의 비율이 컸다. 이렇듯, 시료를 밀도에 따라 분별하여 분석한다면, 전체 시료를 분석하는 것보다 많은 정보를 얻을 수 있을 것으로 기대된다. 밀도에 따른 분별 외에도 Blattmann et al.(2019)은, 점토 광물 분석을 통해 수평적 이동 중 점토 광물과 유기물의 결합이 선택적으로 분리, 치환되는 기작을 밝혀 해양 탄소 교환과 순환에 관한 새로운 아이디어를 제공하였다.
5. 맺음말
퇴적물 트랩을 이용한 연구는 해양 탄소 순환과 심층 해양에서의 탄소 역학을 이해하는 데 있어 중요한 정보를 제공한다. 다층 중장기적으로 계류한 퇴적물 트랩을 이용한 연구는 기후 변화에 따라 생물학적 탄소 펌프의 역할과 심해 탄소 순환이 어떻게 변화하는지에 대하여 이해하고 이를 구체화할 수 있게 하며, 나아가 지구 시스템 모델을 구축하여 미래 탄소 순환을 예측하는 데 있어 보다 정확하고 다양한 정보를 제공할 수 있을 것이다. 비용적, 분석적 어려움으로 지금까지 퇴적물 트랩 연구는 1~5년 정도의 비교적 짧은 기간 동안의 “snap shots”이 대부분이며, 전세계적으로 침강 입자의 방사성탄소동위원소 값 또한 충분한 데이터가 부재한 상황이다. 따라서 재부유 퇴적물의 수평적 이동 기작에 관한 많은 부분들(이동량과 이동 기작, 해양 탄소 순환에의 영향력, 시공간적 변동 등)은 아직 해결해야 할 부분이다. 다양한 기원의 재부유 퇴적물은 밀도나 입자의 크기, 유기물과 금속원소와의 상호 작용, 수층의 단/장기적 역학 등의 영향을 받을 것이기에 데이터의 시공간적 확장과 함께 연구 해역에 대한 다학제적 공동 연구 또한 요구된다(Wakeham et al., 2009; Bao et al., 2019). 향후 국내 연구자들이 꾸준한 관심을 가지고 활약함으로써 전지구적 해양 탄소 순환에 대한 이해를 도울 수 있을 것으로 기대한다.